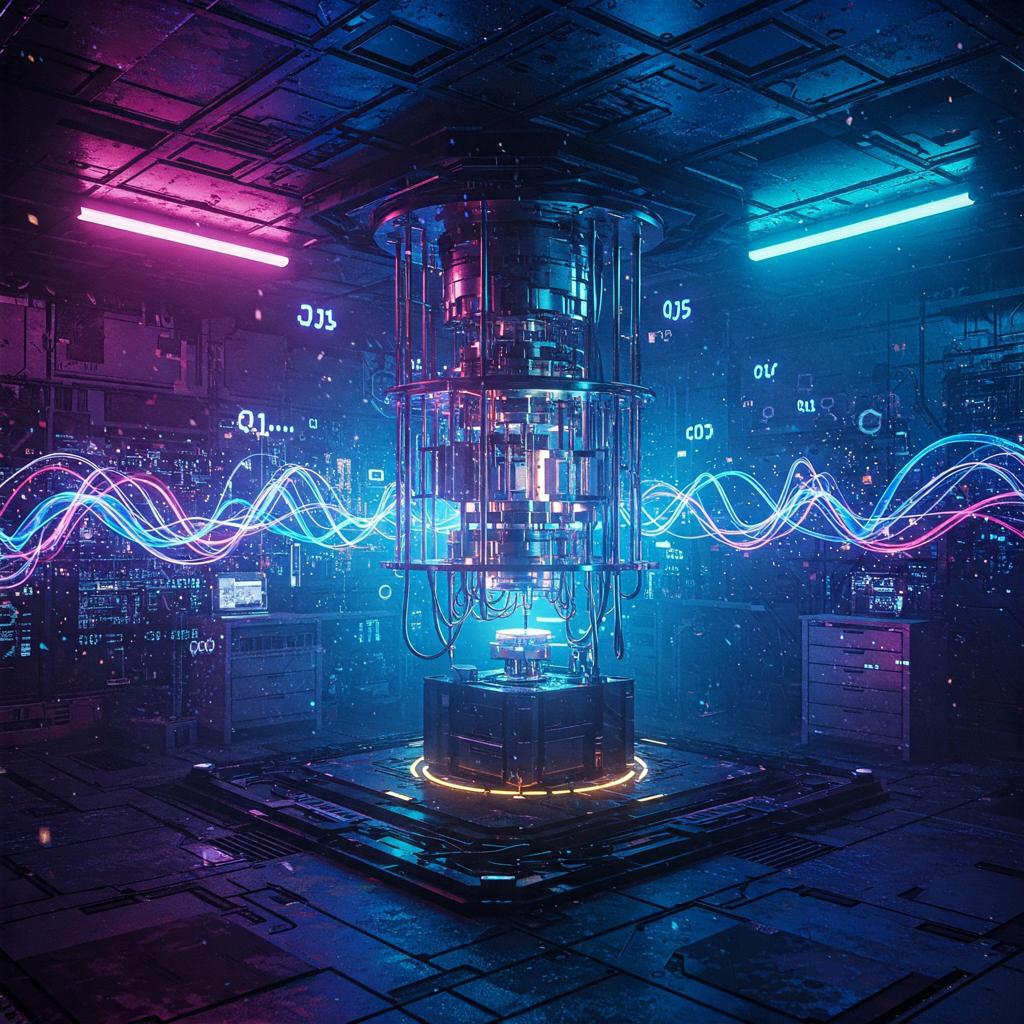
Quantum Computing: Tomorrow’s Digital Core
In the world of technology, a quiet revolution is taking place. It doesn't command as much coverage as the release of the latest smartphone, nor does it receive as much hype as the latest social network craze, but it has the ability to revolutionize our world over the next few decades. We are talking about quantum computers, computers that exploit the strange behavior of quantum physics to process information in ways that our current computers can't even imagine.
But what exactly are these quantum computers? Why are they being funded by billions of dollars by industry giants such as Google, IBM, Microsoft, and governments across the globe? And most importantly, how can this technology revolutionize our daily lives?
In this article, we will take a look into the fascinating world of quantum computers in simple, plain terms. We will see how they work, what problems they can solve, and why they are the future of computers. You don't have to be a mathematics or physics graduate to learn just a little curiosity and openness to new knowledge.
From classical computers to quantum computers
In an effort to understand what makes quantum computers special, we must first understand how the classical computers we use on a daily basis work.
Our computers, phones, and the machines that the net operates on all operate under a fundamental principle: binary information processing. Data within these computers is all wrapped up in strings of 0s and 1s, known as bits. The bit can only have one value at one time: it is either 0 or it is 1, like a light switch that will be either turned on or not.
These bits are physically represented by tiny transistors in processors. When a transistor allows current to pass through, it's a 1; when it blocks the current from passing through, it's a 0. Modern processors contain billions of these transistors, switching on and off billions of times per second, performing logical operations that, when combined, allow us to accomplish anything from sending an email to running complex artificial intelligence models.
This model has worked wonderfully. Moore's Law, that computing power doubles every couple of years or so, has governed technology advancement for several decades. We are reaching the physical limits of this model today. Transistors are now so small that unwanted quantum effects are beginning to make their presence felt, disrupting their normal functioning.
This is where quantum computing comes in. Instead of trying to circumvent such quantum phenomena, quantum computers actually use them. Quantum computers leverage the strange behavior of quantum physics, such as superposition and entanglement, to process information in entirely new ways.
We planned our highways and cities along classical Newtonian physical principles, and now we are discovering a new transportation modality that leverages the precepts of quantum physics not always quicker under every situation, but capable of providing "short cuts" in information space unavailable earlier.
How a quantum computer works
Let's say you need to search a gigantic maze. A conventional computer would need to try one path at a time, backtracking when it reaches a dead end. A quantum computer, on the other hand, because of the peculiar properties it has, could potentially search numerous paths simultaneously.
But how in the world does the magic work?
Quantum computers, unlike classical computers based on electricity and transistors, work by managing subatomic particles or quantum systems under the laws of quantum mechanics. They can employ electrons, photons (light particles), trapped atoms, or other quantum systems.
The hardware of a quantum computer is much different from that of a normal computer. While I'm writing this article on a standard laptop running along quite contentedly at room temperature, quantum computers require extreme conditions. Most must be cooled to close to absolute zero (-273.15°C), colder even than outer space. That's because the quantum properties we want to exploit are extremely sensitive and can be disturbed by the slightest vibration or heat.
The appearance of a quantum computer is no less alien-like. If you've ever viewed pictures of the devices, you can't help but see golden metallic structures that seem to mirror an alien-like chandelier. These are the cooling and insulation systems needed to keep the qubits (the quantum equivalent of a bit, more on them later) under the right conditions to function.
But it's all that hardware complexity for a purpose: to allow quantum computers to leverage two of the key phenomena of quantum physics with no classical analogue:
- Superposition: a bit in a classical computer can only be 0 or 1, but a qubit is in a "superposition" of 0 and 1 at the same time. It is like being 0 and 1 at the same time, but each with a different probability compared to the other.
- Entanglement: it is possible to "entangle" two or more qubits such that the state of one will be immediately correlated with the state of the other regardless of the distance between them. This was called by Einstein "spooky action at a distance" because it seemed to violate relativity's rules.
These two concepts make it possible for quantum computers to perform certain computations in alternative ways compared to ordinary computers. On some very specific problems, that translates to drastic improvements when it comes to speed and capacity.
Qubits: the basis for quantum computing
As stated, the fundamental unit of information in a quantum computer is the qubit (quantum bit). Unlike a classical bit, which can be either 0 or 1, a qubit can be both states simultaneously. This is a difficult concept to grasp as it has no analogs in the macroscopic world we know.
A coin is a good analogy. A classical bit is like a coin that is heads or tails. A qubit is like a spinning coin: it is both heads and tails at the same time, in a way, until we observe it (or 'measure' it, as the quantum physicists put it), at which point it 'collapses' into an actual state.
But this metaphor only goes so far. In the quantum realm, it is not merely a matter of not knowing which state the qubit is in prior to measuring it; the qubit is literally in both states at once, according to strict mathematical laws.
Qubits can be made in a vast number of different ways:
- Superconducting qubits: tiny electrical circuits cooled to very low temperatures, where current flows with no resistance.
- Ion trap qubits: electrically charged atoms (ions) held in position by electromagnetic fields.
- Spin qubits: these use the spin (a quantum property similar to rotation) of electrons or atomic nuclei.
- Photonic qubits: these use quantum states of light particles.
There are strengths and weaknesses to each approach, and it is not yet clear what technology will prevail in the long term.
True potential of qubits is when we put them together. Because of entanglement, 2 qubits can store 4 states simultaneously, 3 qubits can store 8 states, and in general n qubits can store 2^n states simultaneously. A quantum computer with 300 qubits could theoretically store more states simultaneously than there are atoms in the observable universe!
It's this exponential increase in processing speed that is so exciting for some types of problem in quantum computers. But, and it is a big but, we cannot read out the superposed states directly. The act of measuring a qubit collapses the superposition into a single outcome a 0 or a 1 and the original superposition cannot be recovered. The art of quantum programming comes in precisely where we manipulate the probabilities so, when we actually measure, what we observe is what we hope to see with high probability.
The bizarre character of quantum physics
Quantum physics is notoriously un-intuitive. Invented by physicists like Max Planck, Albert Einstein, Niels Bohr, Werner Heisenberg, and Erwin Schrödinger in the early 20th century, the theory accounts for the behavior of matter and energy at subatomic scales in a manner often disconcerting to common sense.
To gain perspective on quantum computers, it helps to familiarize yourself with some of these quantum "quirks":
Heisenberg's uncertainty principle: It is impossible to know both the position and velocity of a subatomic particle with absolute precision. The higher the precision with which we can measure one, the lower the precision to which we can know the other. This has nothing to do with the limitations of our measuring tools, but is a fundamental requirement of quantum reality.
Wave-particle duality: Subatomic particles such as electrons and photons sometimes behave as waves, sometimes as particles, depending upon how we perceive them. This has been attested to by the traditional double-slit experiment, whereby single electrons create an interference pattern similar to waves but register as distinct particles upon measurement.
The wave function collapse: Before we measure it, a quantum system exists in a superposition of possible states described by a "wave function." When we make a measurement, this wave function "collapses" to a particular state. It's as if observation pins down reality.
Schrödinger's cat: We all know about this famous thought experiment that shows the strangeness of quantum superposition. Take a cat and put it inside a sealed box. Connect a device to this cat that would kill it, based on an arbitrary quantum event. Quantum theory informs us that, until one opens the box, the cat is both alive and dead simultaneously. Of course, in our everyday macro life, cats aren't alive and dead at once!
Non-locality and entanglement: As described earlier, entangled particles have the ability to influence each other immediately across a distance, as if "speaking" to each other faster than light. Einstein called this "spooky action at a distance" and considered it proof that quantum theory was incomplete. However, subsequent experiments have confirmed this strange phenomenon of quantum reality.
These features are not mere abstract curiosities: they are exactly the phenomena on which quantum computers rely to operate. Superposition enables qubits to be in many states at once, and entanglement enables these states to be correlated in useful and strong ways for computation.
One last important thing to realize: these quantum characteristics are not exceptions or "glitches" in the universe they are the fundamental rules that everything follows. The macroscopic universe we know, with its "common sense" physical laws, emerges from these quantum behaviors when you have gigantic numbers of particles behaving together. In a sense, everyday reality is an approximation of the more fundamental and strange laws of quantum mechanics.
Why we need quantum computers
You may be wondering: if quantum computers are so difficult to build and maintain, why are we investing billions on building them? Can't we just keep refining normal computers?
The answer is that there are classes of problems which are computationally intractable for classical computers but solvable for quantum computers. It's not a matter of being able to do the same thing faster, but of being able to do the impossible.
Some areas where quantum computers will supposedly dominate are as follows:
Encryption and security: Much of internet security is based on the difficulty of factoring huge numbers into prime factors. It would take a classical computer longer than the universe is old to calculate the prime factors of a number with hundreds of digits. Quantum Shor's algorithm could effectively solve this task in a sufficient amount of time, rendering a great many systems currently in place obsolete. Both an opportunity and a threat, it is an opportunity for a new system based on theoretically invulnerable quantum cryptography but a threat to current systems.
Quantum simulation of systems: Molecules, materials, and chemical reactions are subject to the laws of quantum mechanics. Classical computers go very hard to realistically simulate such systems beyond a point of complexity. Quantum computers, being quantum systems themselves, would automatically be capable of simulating other quantum realities. This would revolutionize drug discovery, materials science, the development of catalysts for clean energy, and much more.
Optimization: Several real-world problems involve the search for the best solution from an enormous number of options. Examples are logistics planning, routing traffic, planning efficient electrical grids, and optimizing financial portfolios. Quantum algorithms like Grover's algorithm or quantum annealing may have a dramatic benefit for these kinds of problems.
Machine learning and AI: Quantum computers could accelerate certain aspects of machine learning, such as finding complex patterns or optimizing neural nets. This would have huge implications for artificial intelligence.
Weather and climate prediction: Atmospheric modeling involves extremely complex chaotic systems with billions of variables. Quantum computers would enable more accurate simulations, which would result in improved weather forecasting and understanding of climate change.
It should be clear that quantum computers will not make traditional computers obsolete for most standard use. You won't have a quantum computer that you would be using to browse websites from the internet or write emails. They are exceedingly specialized hardware that will be solving defined problems out of the purview of common computers.
A better analogy is that of ordinary cars and space rockets. Cars are well suited to everyday use, but rockets are extremely expensive, complex, and specialized but they are the only ones that can take us to space. Similarly, quantum computers are the only ones that can take us to certain otherwise inaccessible "computational spaces."
The challenges of quantum development
Despite all the fuss over quantum computers, the journey to useful, practical quantum computers is fraught with enormous technical hurdles. Some of the largest hurdles scientists and engineers are trying to overcome are:
Decoherence: Quantum systems are extremely sensitive. Interaction with the external world a single photon of light or a tiny vibration will initiate a loss of valuable quantum behavior such as superposition and entanglement. This decoherence effect is one of the biggest problems for quantum computing. It's like building a house of cards in the middle of a hurricane. That's why quantum computers must be encased in super-cold surroundings and isolated from any interference.
Quantum errors: Even with the most complete isolation, quantum systems simply can't avoid errors. In classical computing, we can detect and correct them efficiently, but for quantum systems, it's much harder. It requires a large number of physical qubits to create a single stable "logical" qubit through quantum error correction, making the system many orders of magnitude more complicated.
Scalability: Building a quantum computer with a few qubits is already an impressive feat. Scaling up these systems to hundreds or thousands of qubits required for useful practical purposes exponentially complicates the technical challenges. With each additional qubit, it becomes increasingly more difficult to maintain the system coherent and in control.
Cost and complexity: Current quantum computers are extremely expensive to develop and maintain. They must be housed in specialized facilities, have better cooling facilities, and highly skilled personnel. This limits research and development to large corporations, top-ranked universities, and governments.
Software and algorithms: It is not just a matter of building quantum hardware; we also have to build software and algorithms that are able to take advantage of its power. Quantum programming is a very different paradigm than classical programming, and we are just starting to work in this space.
The "quantum advantage": A key milestone is to demonstrate that a quantum computer will be able to solve a valuable problem more quickly than any ordinary computer. Google claimed in 2019 to have reached "quantum supremacy," but the problem it solved was highly specialized and of limited practical utility. A quantum advantage for valuable real-world problems remains an open question.
Despite these difficulties, the advance continues at a dizzying pace. In the past several years, the number of qubits in the most advanced systems has grown steadily, and the fidelity of the qubits (i.e., error rates and coherence times) has improved dramatically.
The majority of the industry professionals compare what we are at in quantum computing to where the classical computers were in their infancy, when gigantic machines filled the entire room for computing that is easily achievable today with a simple digital watch. We have embarked on just very long marathon, but so vast is the potential at the end of it that it fully justifies the massive investments we see today.
Practical applications of quantum computers
While much of the most revolutionary applications of quantum computers is yet to come, already there are a few areas where progress is beginning to deliver some promising results. Here are some concrete examples of how quantum computers can change some industries in the coming years:
Pharmaceuticals and chemistry: Drug design is a long and expensive process that often relies on trial and error. Quantum computers could precisely simulate molecular interactions, greatly speeding up the creation of new drugs. Several big pharmaceutical companies, including Merck, Biogen, and Pfizer, are already investigating the use of quantum simulation for drug development. For instance, they could be used to create more potent antibiotics against resistant superbugs, or cancer medicines with reduced side effects.
New materials: From room-temperature superconductors to extremely efficient solar panels, much of the breakthrough technology depends on the discovery of new materials with specific properties. Quantum computers could potentially predict the properties of complex materials before they are synthesized in the lab. BMW and Samsung are already putting money into this technology to create improved batteries and more high-performance materials.
Finance: Risk modeling, algorithmic trading in the financial space, and portfolio optimization can all be enhanced with the capability of quantum computing. Goldman Sachs, JPMorgan Chase, and several large banks already are exploring the use of quantum algorithms in these applications. They could potentially help build investment portfolios that balance risk and reward more effectively, or detect fraud in real time by tracking sophisticated patterns in transactions.
Logistics and transport: Vehicle route optimization in fleets, supply chain planning, and logistics planning are highly complex issues that could be assisted by quantum algorithms. Airbus is one of the firms exploring how quantum computers can optimize loading aircraft or air traffic flow. Imagine a logistics system reducing delivery time and expense considerably while limiting environmental impact.
Energy: The power grid is a very sophisticated system that is always having to balance supply and demand. Quantum computers might optimize energy distribution, integrate intermittent renewable sources such as solar and wind power more effectively, and increase overall efficiency. Companies such as EDF Energy are already investigating this field.
Artificial intelligence: Deep machine learning systems require huge levels of computing to train. Some quantum machine learning algorithms can confer huge advantages, especially for computations such as high-level pattern recognition or natural language processing. Google and IBM are heavily investing in the convergence of AI and quantum computing.
Weather and climate forecasting: Current weather and climate models are limited by available computing power. Quantum computers would enable much more detailed and accurate simulations, improving our ability to predict extreme weather and better understand long-term climate change.
It is interesting to mention that the majority of these applications are still in the conceptual or experimental stage. The current quantum computers are small and noisy to solve real-world problems better than classical computers. We are currently in what the majority of experts call the NISQ (Noisy Intermediate-Scale Quantum) era quantum computers with a few dozen qubits and high error rates.
But even now, scientists and businesses are already developing hybrid quantum-classical algorithms that would potentially yield useful advantages even with today's flawed hardware. And with the rapid advancements that we are seeing, most experts estimate that significant practical applications may already begin to emerge as early as the next decade.
The global race to quantum computing
Quantum computer research is not just a science or technology challenge, but a geopolitics issue as well. Quantum research is billions of dollars spent worldwide by corporations and governments, aware that the technology can grant unparalleled economic and strategic advantages to whoever gets there first.
United States: Global leader in the field, with public and private investment. The National Quantum
Initiative Act has put over $1.2 billion into quantum research. Companies like IBM, Google, Microsoft, and companies such as Rigetti Computing and IonQ are at the forefront. IBM already commercialized quantum computers in the cloud, and Google has announced that it has achieved "quantum supremacy" in 2019.
China: Has made the development of quantum computing a national priority with an estimated investment of tens of billions of dollars. The National Laboratory for Quantum Information Sciences is one of the largest quantum research labs in the world. Chinese scientists have also demonstrated quantum advantage in 2020 using a photonic platform. China is the world leader in the build-out of secure quantum communications.
European Union: EU Quantum Flagship is a €1 billion initiative to develop an European "quantum internet." The Netherlands, France, and Germany also spend significantly in big national programmes.
United Kingdom: Launched National Quantum Technologies Program with more than £1 billion investment over a ten-year period, equally shared between public and private investment.
Japan: It has also launched a national quantum strategy with significant investment, with industry through companies such as Fujitsu and NTT.
Russia, Canada, Australia, Israel, and India: Each of them has growing national quantum programs, which are backed by investments ranging from hundreds of millions to billions of dollars.
This global competition also carries strong national security consequences. As reported, quantum computers could potentially undermine much of the encryption technologies that protect government, military, and financial communications. As such, there is a parallel competition to develop new "quantum-safe" cryptography standards before quantum computers are advanced enough to pose a real threat.
In the private sector, competition is equally fierce. Firms are pursuing different paths to qubit technology: IBM and Google are largely emphasizing superconducting qubits; Microsoft is developing more unusual topological qubits; IonQ employs trapped ions; PsiQuantum is building quantum computers based on photons. All have their strengths and weaknesses, and it is too early to say which technology will dominate in the long term.
This global competition is in some ways similar to the space race of the 1960s or the development of the first electronic computers in and after World War II times when revolutionary tech advances reshaped world power. The difference this time is that this competition involves many more national and private players and has the potential to be much more revolutionary for the global economy.
The future of quantum computers
It is difficult to forecast exactly what quantum technology will be like in the next decades, with the pace of innovation and the many technical challenges that lie ahead. Nevertheless, we can outline a few possible directions and milestones along the way.
Short term (5-10 years): We will probably see quantum computers with hundreds or even thousands of qubits, but still with relatively high error rates. These should begin to exhibit useful gains in particular niches such as the simulation of small molecules or particular optimization problems. Most uses will likely be based on hybrid algorithms that merge classical and quantum computing. First concrete commercial applications will likely arrive in areas such as computational chemistry and finance.
In the medium term (10-20 years): We can anticipate seeing the first fault-tolerant quantum computers that have thousands of stable logical qubits as technologies for error correction improve. They may begin to tackle problems of practical significance not accessible to classical computers, such as the realistic simulation of molecules for drug design or new materials. Shor's algorithm might become an actual threat to existing cryptography, speeding up the transition to quantum-safe cryptographic standards. Quantum computers might start being incorporated into cloud infrastructures, making them available to a much broader audience.
In the long term (more than 20 years): This is where speculation starts. Quantum computers with millions of qubits might bring entirely new possibilities. They could revolutionize artificial intelligence, accelerate scientific advances beyond our wildest dreams today, and perhaps even help us figure out some of the universe's hardest problems, from global warming to neurodegenerative illness. Some hypotheses suggest that sufficiently advanced quantum computers could even help us explore some of the universe's most basic mysteries, simply by simulating complex physical systems we can only speculate about today.
And yet, quantum computers will not substitute for classical computers.
Rather, they will be niche tools within a vast computational space. Most of our day-to-day functions will continue to be handled by classical machines, while quantum computers work behind the scenes, processing certain problems that require their particular skill set.
One intriguing possibility is the creation of a "quantum internet" a quantum computer and quantum communications network that could make possible entirely new forms of distributed computing and communications security. Quantum communication, using systems such as quantum key distribution (QKD), already achieves a theoretically unbreakable form of security based on the principles of physics instead of computational power.
Another field with huge potential for research is quantum sensors devices that use quantum effects to take extremely precise measurements. These could transform medical diagnosis, geological exploration, or navigation, being many orders of magnitude more sensitive than the most sensitive classical instruments.
Preparing for the quantum revolution
We are on the cusp of a new era of computation. Quantum computers represent an evolutionary leap as profound as the transition from vacuum tubes to transistors, or even the transition from human computation to the first electronic computers. Like any truly revolutionary technology, their impact will extend far beyond the purely technological, affecting economics, security, science, and perhaps even our understanding of the world.
What does this mean for us today?
For businesses, the time is now to prepare. Even if fully functional quantum computers are still far away, understanding the potential and limitations of the technology will help identify where it will be able to provide value or pose a risk. Areas most exposed to computing, such as pharma, finance, logistics, and energy, already should be factoring in how quantum computing would transform their business models.
For governments and institutions, investment in training and development of quantum capabilities is necessary. Those that become capable of quantum significance the soonest will be considerably strategically advanced. Also needed is preparing for the security implications by implementing quantum-resistant cryptographic standards.
For humans, for students and tech professionals especially, this revolution holds an exciting possibility. The quantum talent pipeline the number of available professionals, including physicists and engineers, mathematicians and quantum software engineers will expand over the coming years with more new job openings and career specialties.
For all of us, as a society, we need to start with the appropriate educated dialogue of what we want to have this technology researched and implemented. As with any powerful technology, quantum computers can be used either beneficially or harmfully. The use of good ethical standards and regulation will be key in enabling the technological development to provide an improved future for all of us.
Last but not least, quantum computers remind us that the frontiers of innovation are still vast and largely unmapped. Despite all our wonderful technological advances, we are only just beginning to discover and understand the basic laws of the universe. Quantum mechanics, in all its strangeness and paradoxes, now gives us a new method of information processing and solving problems that was hitherto unimaginable.
The quantum revolution is on the horizon. It won't happen overnight, and there will certainly be hurdles to overcome first. But so profound is the potential for transformation that we can't afford not to devote our fullest attention and commitment to it. We have no way of knowing what will be learned and created when we initially learn to speak the language of the universe?
Frequently asked questions about quantum computers
Will quantum computers make my existing device obsolete?
No. Quantum computers are extremely specialized devices specially designed to be employed to solve certain problems that are intractable for computers. For almost all but a few specialized problems, classical computers will still be more efficient, less expensive, and reasonable.
Will quantum computers break all our information?
Not immediately, but they could eventually compromise some of the cryptographic systems currently in use. But the cryptography community is already developing new "quantum-safe" algorithms that would be immune even to attacks by extremely powerful quantum computers. Furthermore, quantum cryptography offers new communication protocols that are theoretically unbreakable. There is a race between the development of quantum computers that can break current-day cryptography and the widespread adoption of new security standards.
When will a quantum computer sit on my desk?
Likely never, at least not as we imagine it now. Quantum computers require highly unfavorable conditions (temperatures close to absolute zero for most applications) and tailor-made hardware that make them impractical as consumer devices. Far more probable is that we shall have access to quantum computing power via the cloud, as many applications today use remote servers to perform heavy calculation.
How powerful are quantum computers in current times?
Our largest quantum computers at present are only a few hundred qubits but with still yet to be incredibly small error rates. They can perform some very specialized calculations quicker than classical supercomputers but still too limited and unstable for most practical purposes. We've only just started with this tech, perhaps not unlike the first vacuum tube computers of the 1940s and 1950s.
Can any problem be solved by quantum computers?
No. There are specific types of problems for which quantum computers have significant advantages, such as factoring large numbers, searching unstructured databases, or quantum system simulation. For all other problems, there are little or no quantum algorithm advantage over traditional algorithms. There are also some problems that are inherently hard regardless of the computer type being used.
Should I start learning quantum programming?
If you are already employed in the area of computer science or looking to enter technical areas, studying the basics of quantum computing could be a sound future investment. You can find a variety of tools such as IBM's Qiskit, Google's Cirq, or Microsoft's Q# with simple interfaces to start experimenting with quantum algorithms, even without having very good knowledge of the quantum physics that is behind it. For the majority, though, it will not be required to master quantum programming, any more than it is now required to learn how to write a supercomputer program in order to indirectly enjoy its capabilities.
Glossary of quantum terms
Quantum bit (qubit): The quantum analog of a bit in a classical computer, equivalent to a single bit in digital computers. Contrary to the classical bit that can either be 0 or 1, a qubit can be placed in both positions simultaneously through the principle of superposition. Superposition: Principle of quantum mechanics whereby a system in quantum can exist in two or more than two states at the same instant until measured.
Entanglement: A quantum feature where a group of, or more than two, particles become correlated to a point where one particle's state is not separately defining of the other(s) in a non-distance-dependent way.
Decoherence: The process by which an open quantum system loses quantum behavior (superposition and entanglement) through interaction with its environment.
Quantum error correction: Techniques of detecting and repairing quantum computation error caused by decoherence and other types of noise.
Quantum gate: One of the building blocks of a quantum circuit operating on the state of one or more qubits, analogous to the logic gates of classical digital circuits.
Shor's algorithm: A quantum computation, developed by Peter Shor in 1994, to break large integer factorization exponentially more efficiently than the best known algorithms on a classical computer, that may pose a threat to a large number of cryptographic systems today.
Grover's algorithm: A quantum algorithm that can search for an element in an unstructured database with a quadratic advantage over classical algorithms.
Quantum supremacy (quantum advantage): The point beyond which a quantum computer can solve a problem that is effectively infeasible for any classical computer.
Fault-tolerant quantum computer: A quantum computer with sufficient error correction to perform arbitrarily long computations with high fidelity, even when there are errors in the underlying physical qubits.
Post-quantum cryptography: The development of cryptographic protocols that would be secure against attack by very powerful quantum computers.
Quantum annealing: An application of quantum computing that leverages quantum phenomena to find global minima in hard optimization problems.
Sources for further reading
If you have been sparked into interest by this article and would like to learn more about quantum computers, here are some useful resources to take on your journey of discovery:
Best-selling books:
- "The Quantum Computer: The New Frontier of Computing" by Artur Ekert and Antonio Acín
- "Quantum Computing: An Introduction Without Mathematical Formulas" by Chris Bernhardt
- "The Elegant Universe" by Brian Greene (has sections on quantum mechanics)
Online courses:
- "Quantum Computing for Everyone" on edX, taught by MIT
- "Quantum Computing. Less Formulas - More Understanding" on Coursera
- "Quantum 101" from QWorld, a non-profit organization for quantum outreach
Platforms for experiments:
- IBM Quantum Experience: provides access to real quantum computers through the cloud
- Microsoft Quantum Development Kit: the Q# programming language and simulators • Google's Quantum Playground: a browser-based quantum simulation platform
Websites and blogs:
- Quantum Computing Report: news and analysis of the industry
- Quantum Frontiers: blog of the California Institute of Technology
- Quantum Computing Stack Exchange: community Q&A site
Videos and documentaries:
- YouTube channels such as Quantum Computing Italy, Veritasium, and PBS Space Time contain excellent educational videos
Remember that quantum computing is a constantly evolving area, so always look for recent resources to stay current on the newest breakthroughs. Although some of the language may seem intimidating at first, with a bit of curiosity and perseverance, anyone can begin to understand the fundamental principles of this amazing technology.